Newsletter #6 – September 2020
Download pdf version here. Previous newsletters: Newsletter #1, Newsletter #2, Newsletter #3, Newsletter #4, Newsletter #5
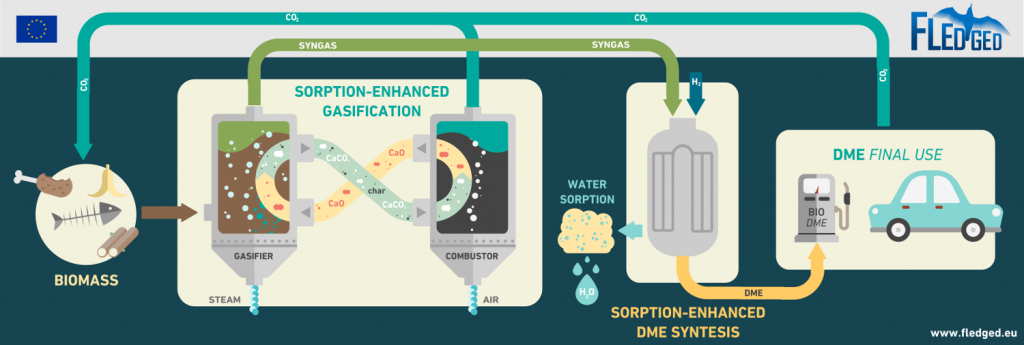
FLEDGED activities on biomass gasification are completed and in the last months the work focused on Sorption-Enhanced DME synthesis …
- TRL5 experimental testing of the complete SEDMES operating cycle has been concluded at TNO, supported by SEDMES cycle modelling for operation mode design and optimization.
- Catalyst development for direct DME synthesis in presence of sorbent for Sorption-Enhanced synthesis has been performed at ICP-CSIC laboratories in Madrid.
- A 2D + 1D heterogeneous dynamic modelof the reactor tube for design optimization has been successfully developed by POLIMI, validated and applied for defining operating parameters on thermal behavior and DME yield performances of the reactor.
More details and short presentations about all the activities of the partners are available on the intermediate workshop dedicated webpage.
TRL 5 demonstration of Sorption-Enhanced DME Synthesis and modelling activities on cycle optimization (TNO)
Cycle design for six columns SEDMES unit with implemented pressure equalization system meets the following boundary conditions: 1)continuous feed stream; 2)continuous DME production; 3)continuous purge stream. Six step pressure swing adsorption (PSA) cycle (total 45 minutes) consists of:
- ADS adsorption step (DME production);
- PEQDN pressure equalization down (depressurisation);
- BD blowdown (depressurisation);
- PURGE purge (regeneration);
- PEQUP pressure equalization up (pressurization);
- REP repressurisation (pressurization).
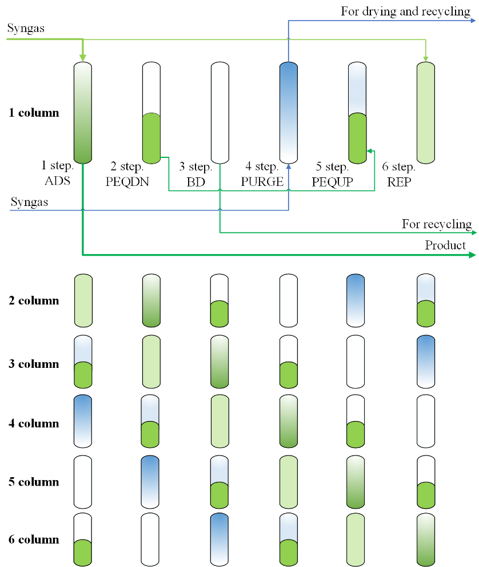
The data from the multiple simulations showed Pareto fronts, typical for PSA systems, for the defined key performance metrics C-DME, i.e. the single pass carbon selectivity to DME, and C-CO2, i.e. the selectivity towards CO2, and productivity, which allows to tune the process performance towards selectivity and productivity targets. In good agreement with experimental results, high carbon selectivity to DME can be obtained (over 95%). Similarly, the carbon selectivity to CO2 can be reduced to less than 1%, simplifying the downstream separation. However, both come at a cost in terms of productivity. Maximum productivity can be obtained for carbon selectivity to DME of over 80%. The average productivity for a single SEDMES tube is 0.17 kg hr-1.
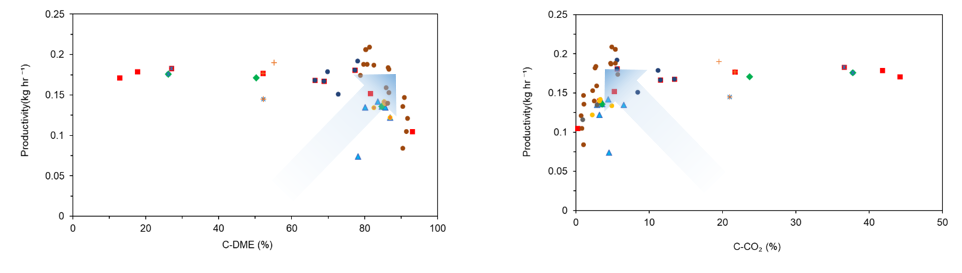
Using the developed mathematical model it is possible to predict which process happens in the single reactor and how the components are distributed within the length of the reactor. This allows to select optimal process conditions in order to achieve maximal yield and purity of the product. For experimental validation, a 6 reactors test unit was operated at the same condition as the simulations, and the composition of the product was sampled in time. Using TNO’s multi-column SEDMES reactor setup, the TRL 5 validation experiments were performed in the first half of 2020. The multi-column system allows to test complete cycle designs, e.g. including pressure equalisation steps. Besides model validation experiments at different temperatures in the range of 230-250 °C, specific conditions included CO-rich and CO2-rich feed gas, up to a pure H2-CO2 feed gas.
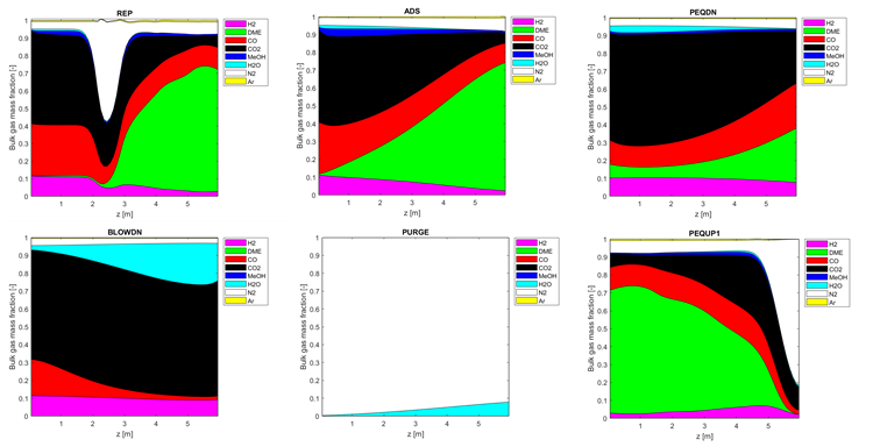
Also different cycle timings were evaluated experimentally. Overall, material stability has been validated by testing for more than 500 full SEDMES cycles. As shown, the experimental results are in close correlation with the simulated results. Therefore, the model can be used in the future prior to experiments to adjust the process parameters in accordance with a current target.
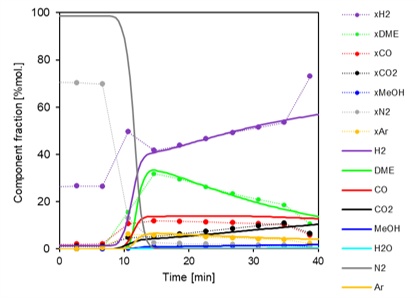
Catalyst development for indirect and direct DME synthesis (ICP-CSIC)
The Group of Sustainable Energy and Chemistry from the Instituto de Catálisis y Petroleoquímica, from CSIC, developed a series of Cu/ZnO based catalysts for the synthesis of methanol from CO2-rich bio-syngas and acid catalysts for the production of DME from methanol. The aim was to find the optimal catalyst composition to work under the optimum reaction conditions Sorption Enhanced DME Synthesis (SEDMES), performing the reactions with a physical mixture of one of the methanol catalysts and commercial g-Al2O3 and employing a sorbent zeolite, 3A type. The Cu/ZnO/Al2O3 catalysts were doped with ZrO2 and Ga2O3 (see Table below). The catalysts were synthesized by coprecipitation of the metallic salts under controlled pH and evaluated in a fixed bed reactor using a syngas with CO2/CO-ratio equal to 1.9.
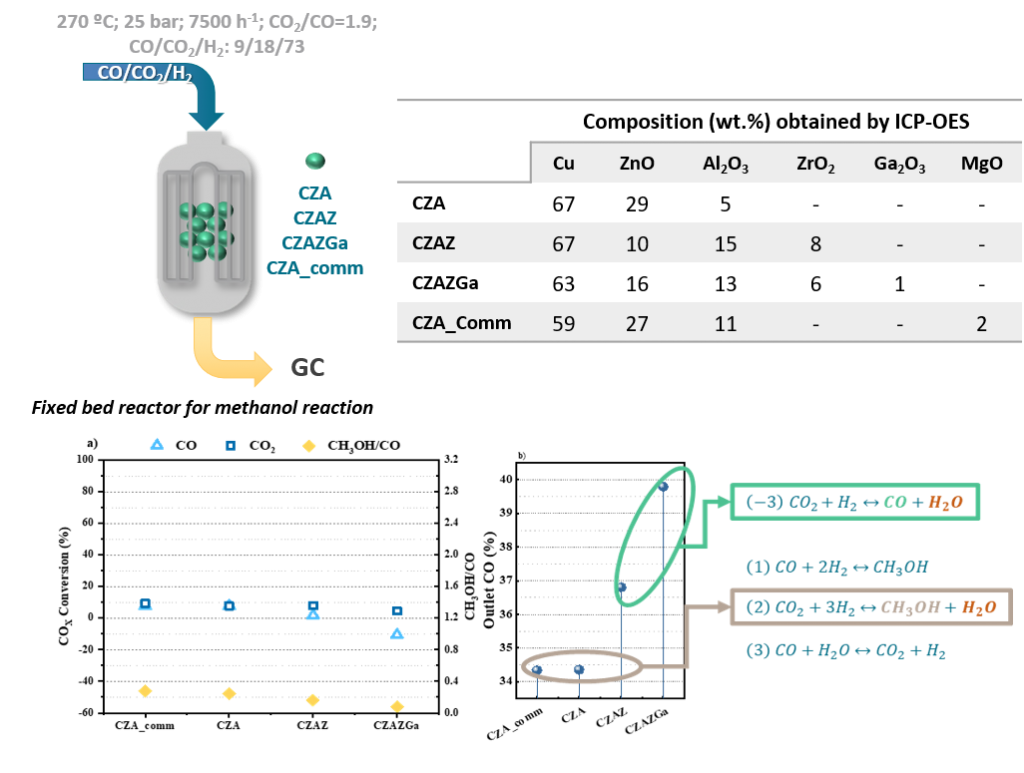
- Both CZA_comm and CZA record the highest CO and CO2 conversions.
- Doping the catalysts with ZrO2 decreases CO conversion to ca. 1.6 %, without affecting CO2 conversion.
- Ga2O3 doping (CZAZGa) increases the CO/CO2 ratio of the syngas (CO conversion of -10.6%)
- Negative conversion values indicate that CO is formed during the process via the r-WGS reaction, which is promoted by the addition of ZrO2 and especially Ga2O3 to CZA.
The Direct Synthesis of DME (DDMES) form biomass-derived CO2-rich syngas over a catalytic bed comprising a mixture of the Cu/ZnO catalysts and g-Al2O3 was studied at TNO facilities. The effect of in situ water removal for the synthesis of DME was also studied under the same conditions using zeolite 3A as water sorbent. This approach is known as Sorption Enhanced DME Synthesis (SEDMES). Figure 2 illustrates how experiments were recorded. A catalytic bed containing the Cu/ZnO catalyst, g-Al2O3 and zeolite 3A was used. The pre-breakthrough region, when zeolite 3A is capable to remove the H2O produced, accounts to the SEDMES. After zeolite 3A saturation, the configuration is for the DDMES.
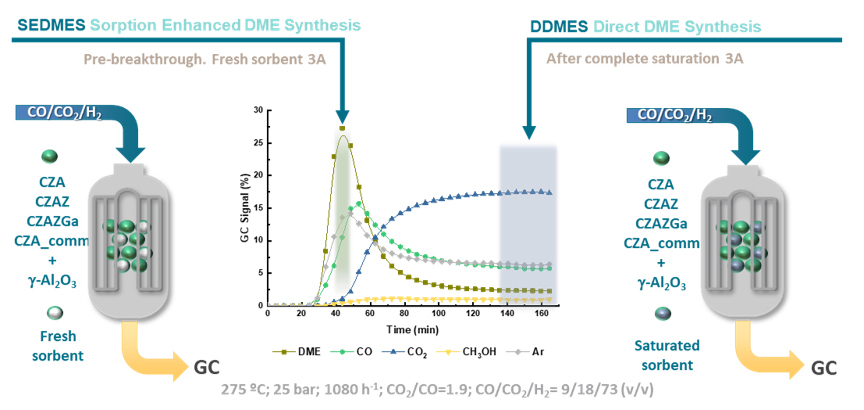
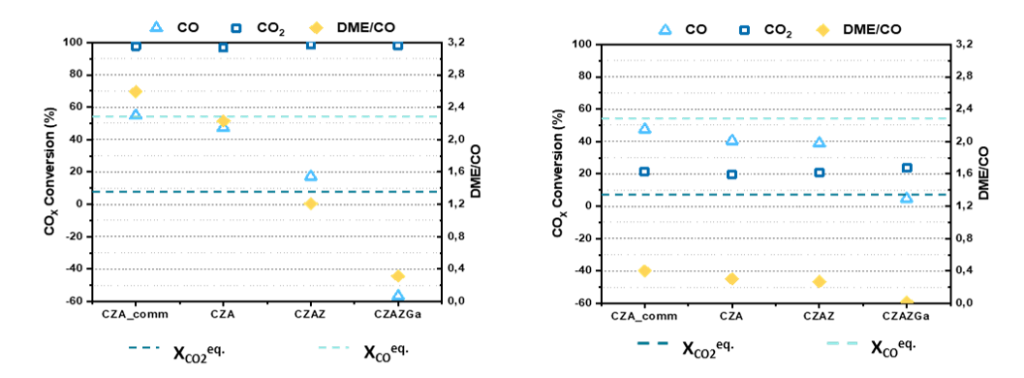
- The in situ removal of H2O during the SEDMES process shows a marked effect, displaying higher CO and CO2 conversions than conventional DDMES.
- Non promoted CZA catalysts (CZA_comm and CZA) display higher CO2 conversions and DME production than the promoted catalysts in both SEDMES and DDMES regimes.
- The effect of ZrO2 and Ga2O3 doping (CZAZ and CZAZGa), especially Ga2O3, is more obvious in the SEDMES process.
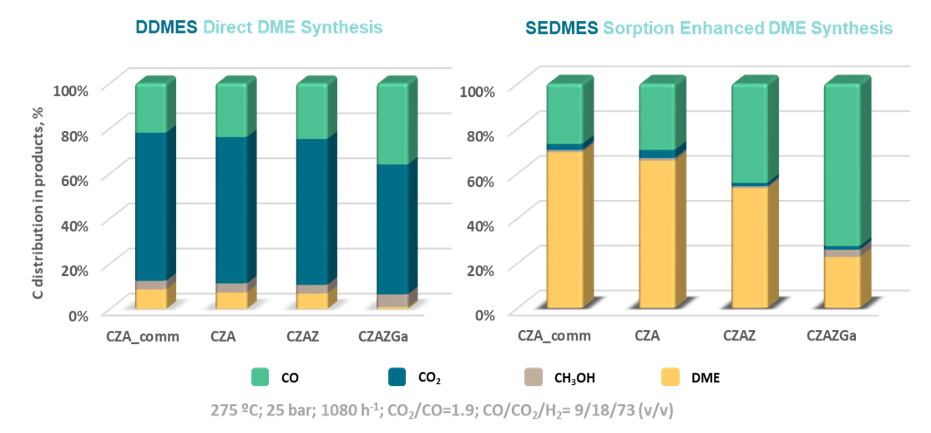
As clearly shown in Figure 4, H2O removal during the direct synthesis of DME from CO2-rich syngas result significantly higher DME production, especially over non-promoted Cu/ZnO catalysts. However, the actual promotional effect is also affected by the catalyst, thus:
- Water removal during SEDMES over CZA catalysts promotes methanol production and dehydration reactions
- Water removal during the SEDMES over catalysts with high r-WGS activity promotes CO production
In summary, the promotional effect of the SEDMES approach for the direct synthesis of DME from biomass-derived CO2-rich syngas has been demonstrated experimentally in the frame of FLEDGED project.
2D + 1D heterogeneous dynamic model of the SEDMES synthesis for reactor design and optimization (POLIMI)
The development of the mathematical 2D+1D dynamic model for SEDMES multitubular fixed bed reactor has been completed. The model has been implemented in gPROMS commercial language for the numerical solution and has been validated by a comparison with bench scale reactor experimental data obtained in the TNO facilities in Petten. The model shows the ability to capture the experimental dynamics of the outlet composition of reactants (CO, CO2) and products (CH3OH, DME) and also to describe the temperature trajectories in different points along the axial reactor coordinate.
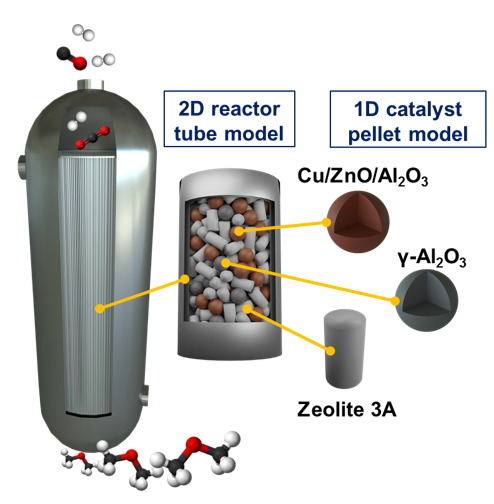
From the local trajectories the envelope of the maximum temperatures along the axial coordinate is obtained. Comparison with the maximum values of temperature measured at the different axial locations during the adsorption/reaction step shows that the model is able to capture the catalyst temperature stress suffered during SEDMES operation, which is a key parameter in reactor design.
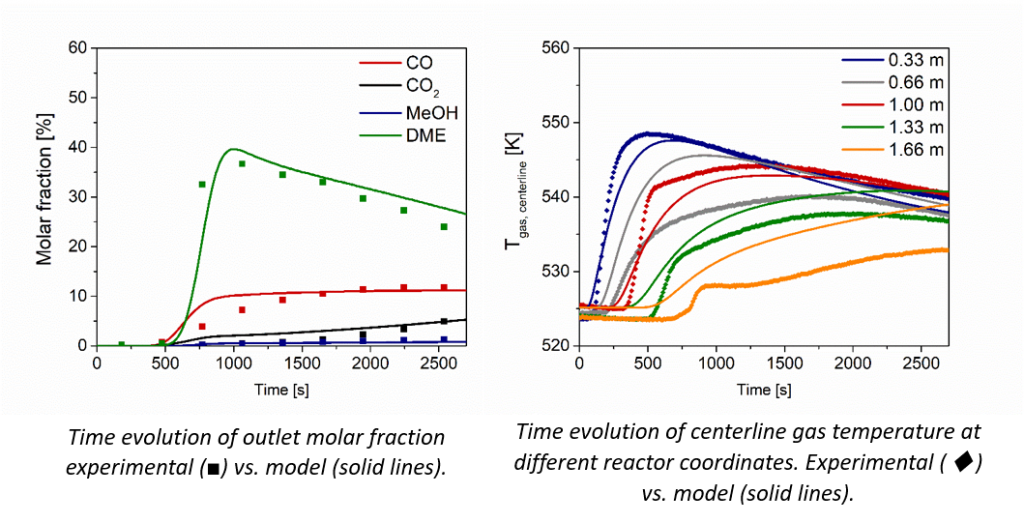
The validated model has been used for the analysis and rational design of the industrial scale SEDMES reactor, focusing in particular on the DME yield performances and the thermal management of the exothermic process. The envelope of maximum temperatures achieved along the axial coordinate shows that catalyst thermal stresses in the hotter inlet zone of the reactor slightly increase with the CO content in the with due to faster kinetics of the DME production reactions.
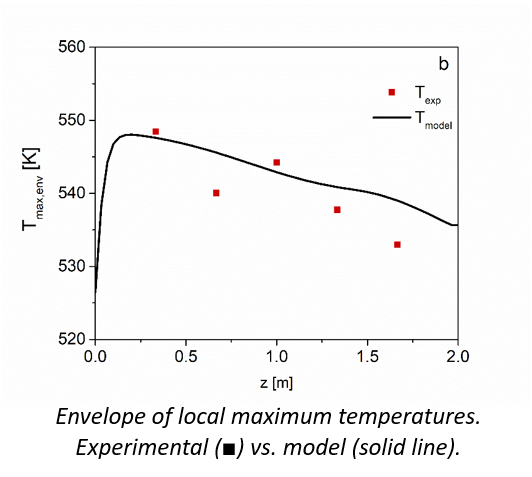
However, thanks to the dilution effect associated with the large amount of adsorbent material (catalyst:adsorbent=1:4 w/w), the maximum bed temperature keeps well below the limits reported in the literature (573 K) to preserve the CZA catalyst stability. Accordingly, larger tube diameters (up to 46.6 mm) than in conventional DME direct synthesis reactor can be adopted.
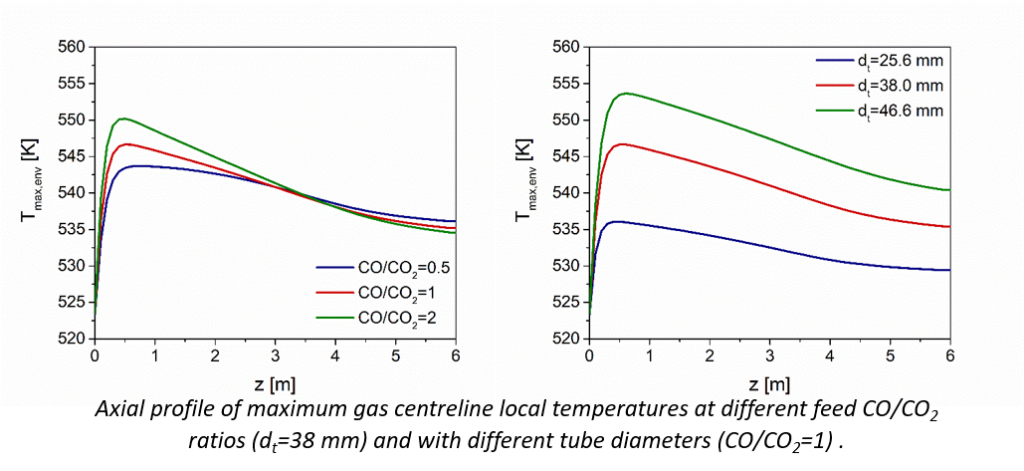
Scientific publications
Several publications on SEDMES and SEG technologies have been released. They are fully accessible from the dedicated webpage.