Newsletter #4 – June 2019
Download pdf version here. Previous newsletters: Newsletter #1, Newsletter #2, Newsletter #3
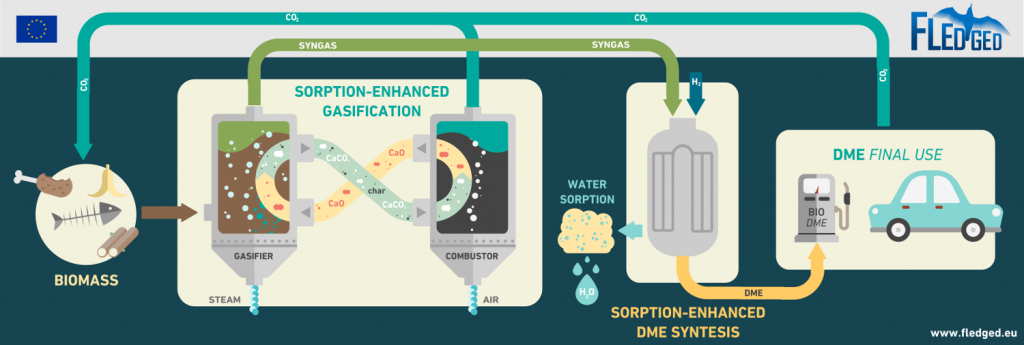
FLEDGED project reached its second milestone
FLEDGED project is entering now in its conclusive step and it is time to brainstorm before the final sprint. Some results are already available and activities are running fast. The fifth project meeting of the FLEDGED consortium was hosted by Lappeenranta University of Technology. The participants visited the impressive UPM Kaukas Biorefinery and power plant, where renewable diesel from residual biomass is produced.
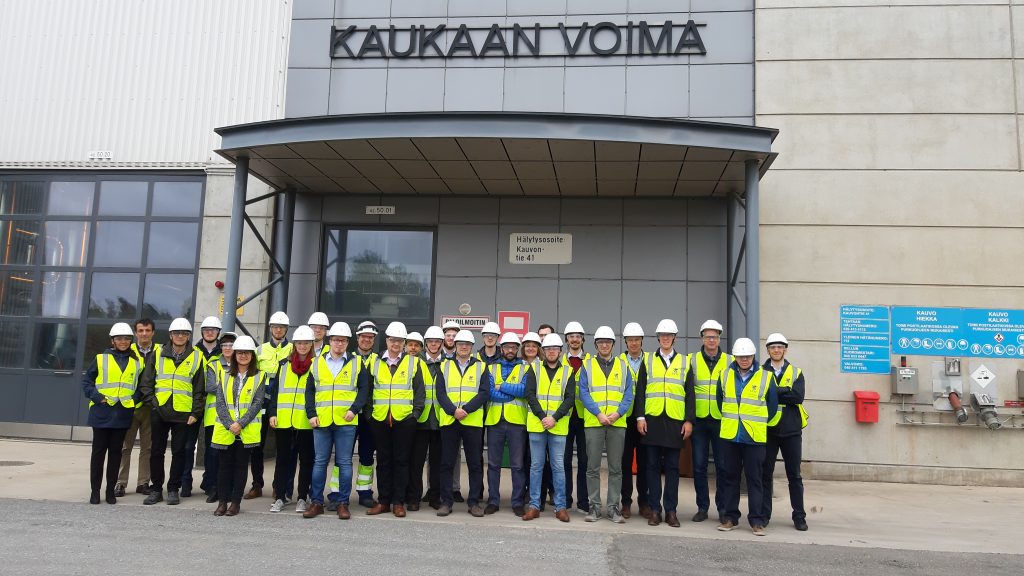
The results obtained during the last months and the ongoing work of the partners are presented in the next pages:
- Gasification tests in the pilot dual fluidized bed at University of Stuttgart and in the bubbling fluidized bed at CSIC-ICB
- Experimental activities on SEDMES in the pilot rig at ECN.TNO and catalysts characterization at CSIC-ICP
- Dual fluidized bed gasifier CFD modelling activities at LUT and DME synthesis reactor simulation by POLIMI-LCCP
- Complete process modelling and economic assessment (POLIMI)
- Risk analysis considering process hazards identification and materials characterization (INERIS)
- Environmental LCA, air quality and socio-economic impact assessment (QUANTIS)
- Business cases for different plant size and feedstocks (Econward, Sumitomo FW and FRAMES)
Experiments in the pilot scale facility at USTUTT
In the 200 kWth dual fluidized bed facility at USTUTT, tests of the sorption enhanced gasification (SEG) of wood pellets have continued. Experiments were conducted at different gasification temperatures and different steam-to-carbon ratios. It was possible to modify the M-module in a range between 12 and 1, showing the flexibility of the process. Also the target value for DME production (M=2) has been reached. Some of the results from these tests are presented in the diagrams below. The experimental experience showed that the process can be operated stably in the pilot scale facility at gasification temperatures between 650 °C and 775 °C. Syngas composition and M-module strongly depend on gasification temperature due to the influence on the equilibrium of the carbonation reaction, which influences the amount of CO2 removed from the syngas.
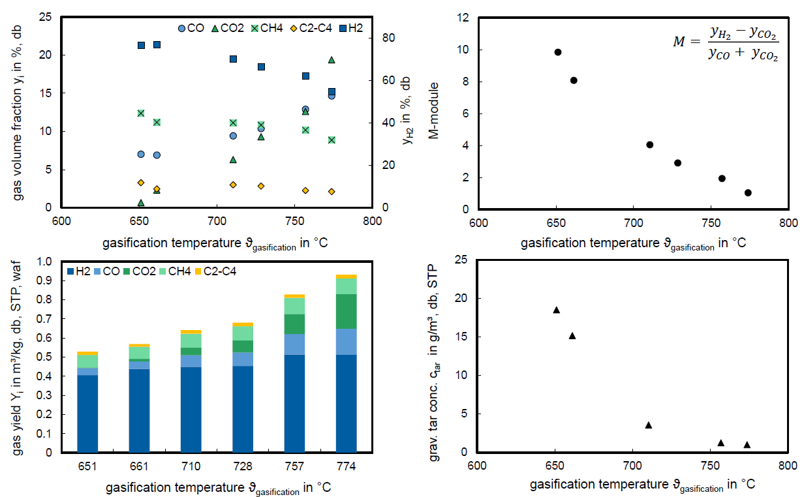
Syngas composition, M-module, gas yield and tar concentration for Sorption-Enhanced gasification with wood pellets between 650 and 775 °C (S/C=1.5).
No significant influence of S/C ratio on standard gas composition and yield has been evidenced, while tar concentration decreases with increasing S/C. In addition to the experiments with wood pellets, the first experiments with biogenic waste material from the Econward plant have been successfully conducted. The abovementioned results have been presented at the European Biomass Conference (EUBCE 1019) in Lisbon. The presentation is available for download on the website.
Experiments at the ICB-CSIC 30 kWth bubbling fluidized bed gasifier
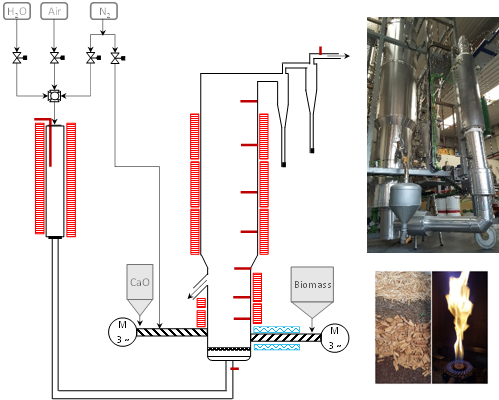
Configuration of the 30 kWth bubbling fluidised bed reactor at ICB-CSIC.
In the 30 kWth bubbling fluidized bed (BFB) facility at ICB-CSIC, the testing of the sorption enhanced gasification (SEG) using six different biomass feedstocks has been recently concluded. Experiments using different gasification temperatures, sorbent-to-biomass and steam-to-carbon ratios have been conducted, which allowed assessing the influence of each operating variable independently. Gas yield has been found to be mainly linked with the char conversion reached in the reactor, which is influenced by the temperature, the solid residence time and the type of biomass. No significant effect of the steam-to-carbon ratio has been found in the content of H2, CO, CO2 and CH4 in the syngas (and so on the M-module) when keeping the temperature and the sorbent-to-biomass ratio constant. The M-module is driven by the gasification temperature and the sorbent-to-biomass ratio by means of the CO2 separated from the gas phase, and the optimum value for DME production (M=2) has been reached for most of the biomass tested. The biogenic waste material from the Econward plant resulted to be among the most reactive feedstocks tested, resulting in the largest gas yields (i.e. up to 1.6 Nm3/kgwaf biomass). However, in a temperature range of 650-680ºC this waste biomass could lead to the largest tar yields compared to other biomasses (see the figure). The combustion of the unconverted char coupled to the calcination of the carbonated sorbent has been also studied in the BFB facility at ICB-CSIC.
Experiments using different char/sorbent ratios, air excess amounts and temperatures have been conducted for chars coming from two different biomass feedstocks. Combustion and calcination efficiencies higher than 98 % and 94 %, respectively, have been reached when operating the reactor at 860°C, being the formation of polycyclic aromatic hydrocarbons (PAHs) being the main issue to be solved. All the results obtained at the ICB-CSIC facility will be included in the public deliverable 2.5 available soon in the dedicated section of the website.
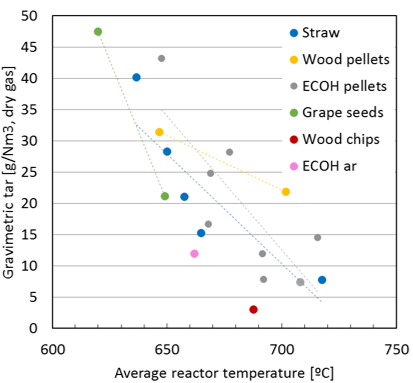
3D modelling of large scale SEG process (LUT)
LUT has continued activities with Sorption-Enhanced Gasification (SEG) modelling. Recently a new 3D model for connected SEG reactor system has been developed.
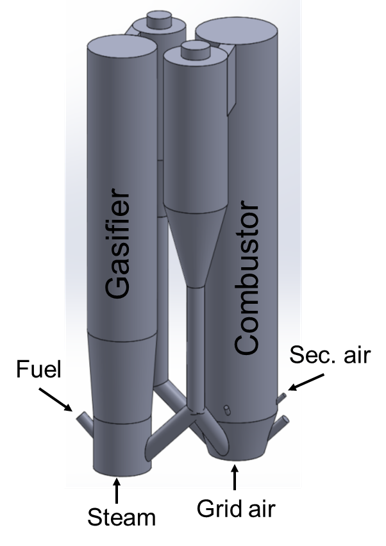
This 3D model is a steady state semi-empirical engineering model for CFB reactors. The inhouse model combines modelling of all major heterogeneous and homogenous reactions, fluid dynamics of gas and solids, and heat transfer. New coupling scheme allows to connect multiple CFB reactors with various solid and gas stream connections, which provides an option to study different SEG process configurations. In this preliminary simulation case wood-based biomass was used as a fuel with thermal power of 100MW. Both reactors with height of 20m and approximately identical in diameter were investigated. Target producer gas module was achieved (i.e. M»2) with producer gas concentrations (vol-%,dry): H2=62.5%, CO=11.6%, CO2=12.2% and CH4=10.5%. Submodel validation work is ongoing providing more reliable modelling results from upcoming simulation studies.
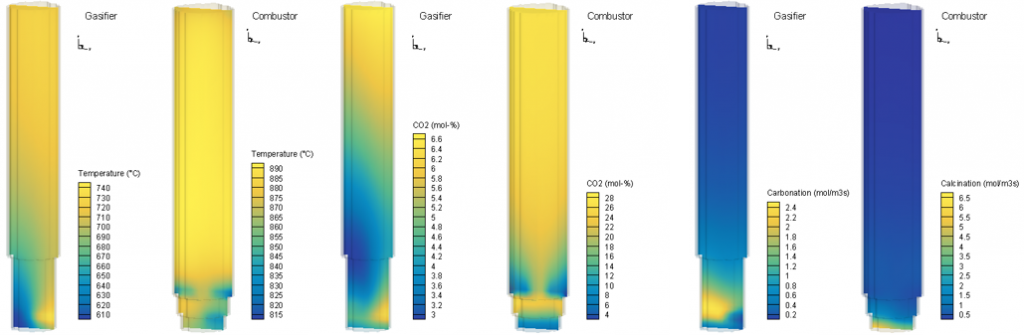
Sorption-enhanced DME synthesis (SEDMES) at ECN.TNO
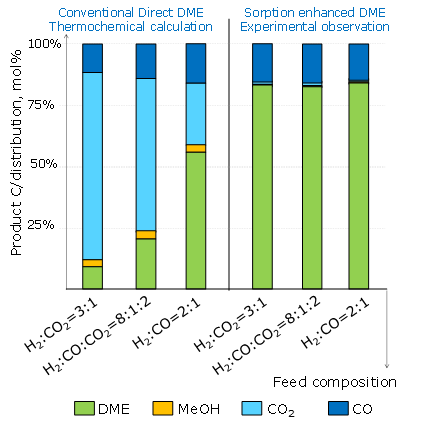
ECN part of TNO published on the Chemical Engineering Journal a review paper on the outlook of separation enhanced processes. Summarising over 120 literature references, it has been concluded that steam separation enhancement is a promising process intensification technology especially for CO2 utilisation. The feasibility of reactive steam adsorption processes, such as sorption-enhanced DME synthesis (SEDMES) require high working capacity, heat management as is currently being addressed in the FLEDGED project. Finally, it became clear how progress in process development requires combination of theory and experiments. Preliminary testing (TRL3) in the laboratory at ECN.TNO has led to a dataset (>300 SEDMES runs) of performance, in terms of DME yield and DME productivity, as a function of operating parameters. The potential operating window has been defined and a suitable combination of catalyst for direct DME synthesis and zeolite water adsorbent. All smart targets for the small-scale testing were successfully met:
- COx content halved compared to steady-state/equilibrium
- Fully flexible with respect to CO-CO2 ratio in the feed
- Single pass CO2 production below 5%
Experiments are now started at the bench-scale (TRL4) setup, using a single column of 2 m for testing the transient behaviour of the SEDMES reactor column. Combining theory and experiments, modelling efforts have continued. The dataset that was obtained in the TRL3 testing is currently being used to validate the SEDMES reactor models at ECN.TNO as well as at POLIMI. To further intensify cooperation, POLIMI PhD candidate Simone Guffanti has moved to ECN.TNO in Petten for the first half of 2019, where he focuses on aligning the heat balance modelling with bench-scale experiments as well as intra-particle mass transfer phenomena. Currently, the modelling efforts at ECN.TNO are directed towards optimising the SEDMES cycle design in terms of DME productivity.
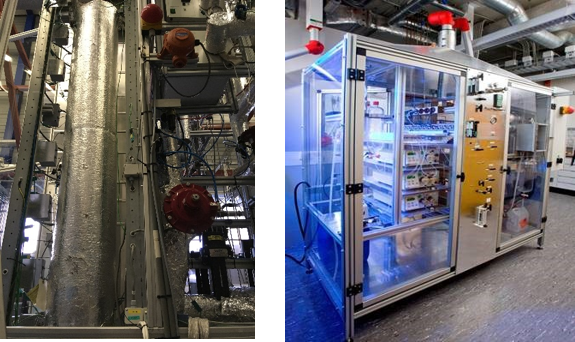
Catalyst development at CSIC-ICP
The CSIC Institute of Catalysis and Petrochemistry, developed two series of catalysts: one series for the methanol synthesis from bio-syngas and one for DME synthesis from methanol dehydration. The of this work was to find the optimal reaction conditions to obtain the direct synthesis of DME from bio-syngas by performing the reactions with a physical mixture of the two series of catalysts. The physicochemical properties of the synthesized materials were thoroughly analyzed.
Methanol production from bio-syngas
A series of Cu/ZnO/Al2O3 catalysts with different Cu loading was studied, including the commercial Cu/ZnO/Al2O3 catalyst for methanol production. The effect of the reaction pressure, temperature, contact time and bio-syngas composition was analyzed, as well as the use of promoters: Pd, Zr and Ga. Here we show the results of the experimental matrix developed to study the reaction conditions using the commercial catalyst.
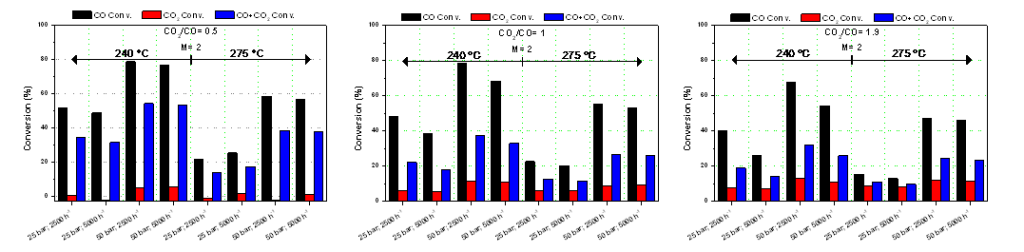
DME production from methanol
The experimental results for synthesis, characterization, and catalytic performance of supported HPA, HZSM5 and g-Al2O3 catalysts for methanol dehydration are presented. Heteropolyacids are very active for methanol dehydration reaction. When supported onto inorganic solids they show very high methanol conversion to DME at temperatures as low as 140 ºC with 100% selectivity to DME under undesired hydrocarbon by-products. HZSM5 and g-Al2O3 are used as acid benchmark catalysts to compare the methanol dehydration performance with supported HPA catalysts.
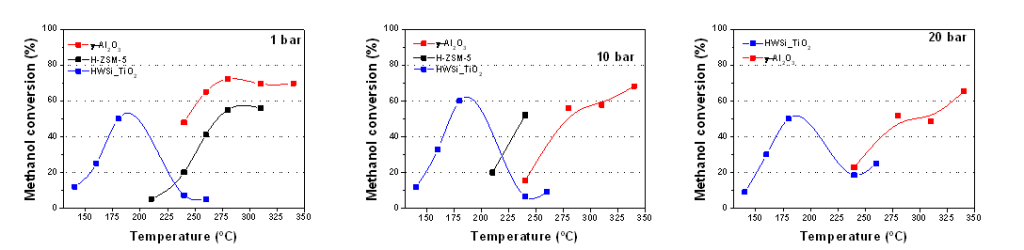
DME synthesis reactor modeling (POLIMI-LCCP)
The development of the 2D reactor models for the direct DME synthesis has been completed at the LCCP group of POLIMI: simulation codes of a conventional reactor and a SEDMES reactor have been implemented in gPROMS software environment for the process analysis. The conventional multitubular reactor behavior has been described using a heterogeneous steady state single-tube model. Governing equations consist of i-species mass, energy and momentum balances of the fluid phase written in 2D cylindrical coordinates, coupled with i-species mass and energy balances for the catalyst phase accounting for concentration gradients (1D) in an isothermal catalyst pellet. This model has been used as a tool for an extensive parametric analysis aimed to identify the best operating conditions (temperature, pressure, space velocity and composition) and to design the reactor (tube diameter and length) in order to maximize the DME productivity and controlling the temperature hot-spot. The analysis includes also a detailed study of the possible different configurations (hybrid, mechanical mixture, core@shell) of the two catalysts present in the DME direct synthesis process (methanol synthesis and methanol dehydration catalyst). The 2D dynamic SEDMES reactor model has also been developed and implemented in gPROMS and it is now ready to make predictions on the reaction/adsorption behavior of the process. For this purpose, the POLIMI PhD candidate Simone Guffanti moved to ECN.TNO for a six months internship. The focus of the cooperation is the SEDMES model assessment achieved through an experiment/model comparison and the analysis of the mass and heat exchange phenomena inside the bench-scale reactor. The assessed SEDMES model will be used for the rational design of the SEDMES reactor and comparison of the performances with those obtained with the conventional reactor model.
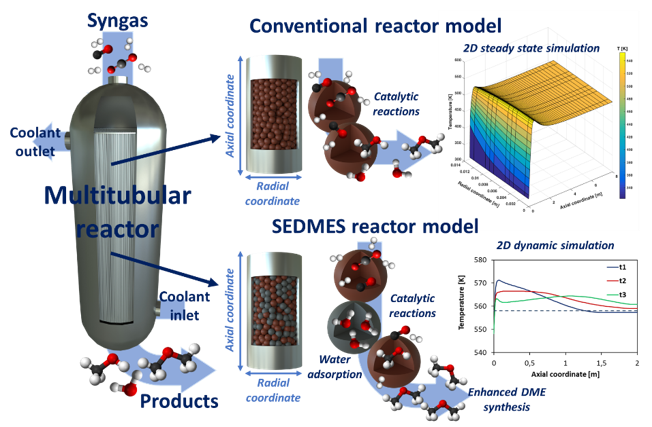
Preliminary techno-economic analysis of the complete FLEDGED plant released by POLIMI
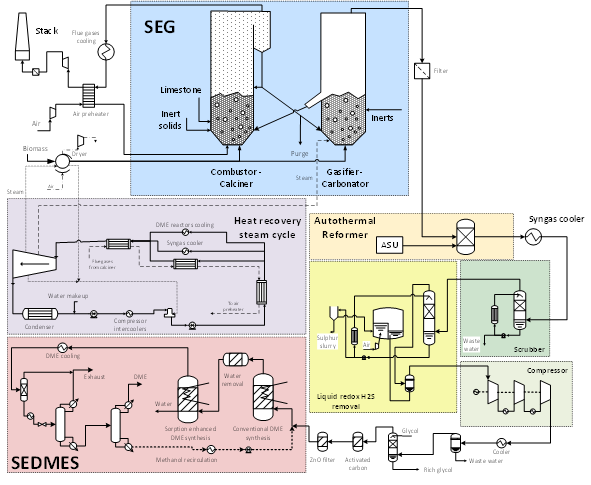
POLIMI developed an Aspen Plus model to calculate the heat and mass balances of a reference complete FLEDGED plant, including: i) a biomass drying and preparation unit, ii) the SEG process, iii) the syngas cleaning units for the removal of tar (OLGA), soluble contaminants (water scrubber), bulk sulfur removal (liquid redox process) and residual contaminants and H2S (activated carbons and ZnO bed), iv) syngas compression, v) DME reactors and vi) DME separation unit.
The model allowed calculating the key performance indicators such as the biomass-to-DME conversion efficiency and the electric efficiency. The calculated overall “equivalent” cold gas efficiency CGEeq (i.e. taking the primary energy credits/debits associated to the electric balance) is in line with those reported in the literature for other biomass to DME processes based on air-blown gasification systems. An economic analysis of a plant with 100 MWLHV of biomass input was also performed. With a biomass unit cost of 125 €/t, the calculated DME cost ranges between 46 and 55 €/GJ, largely associated to the cost of biomass (57%) and to the investment cost (40%).
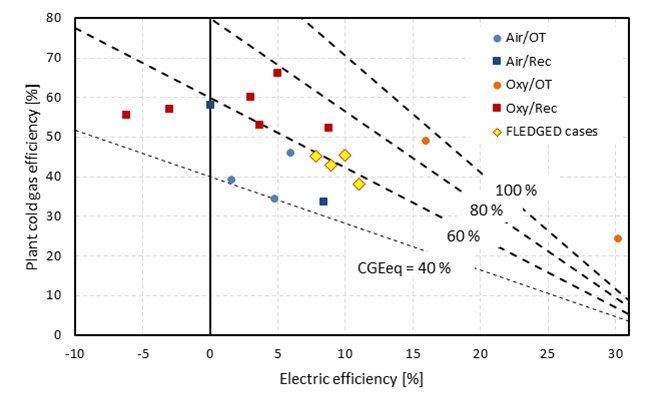
This preliminary techno-economic analysis allowed identifying the critical plant units and the governing process parameters. Lessons learned will be used in the second half of the project to explore solutions capable to increase the economic competitiveness of the process.
The preliminary techno-economic study with a detailed description of the model and of the results obtained is available as public deliverable on the project website.
Risk assessment in the FLEDGED project (INERIS)
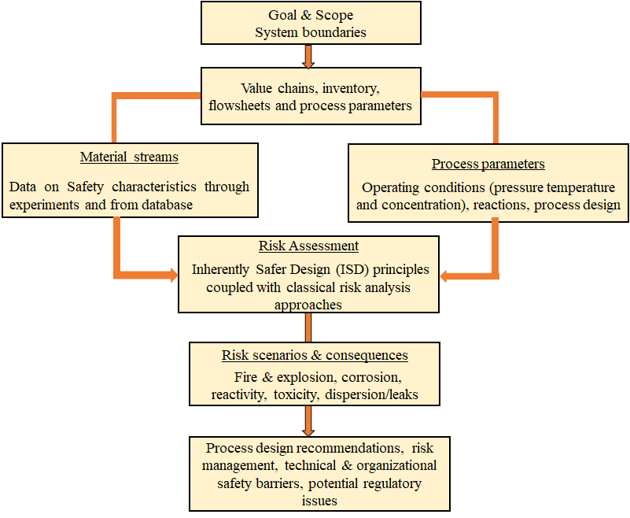
Increased public awareness of industrial safety issues and regulatory demands as response to accidents has led to the integration of risk assessment studies at the early stages of innovative process design and development. Risk assessment carried out in the FLEDGED project aims at eliminating or minimizing identified hazards from the process rather than incorporating add-on controls and safety barriers at a later stage. A stepwise methodology is implemented to study the risks related to process steps and materials all along the value chain, making use of Inherently safer Design (ISD) approach for the safe and sustainable development and deployment of the FLEDGED technology. One of the tasks in progress is the safety characterization of the material streams for the physico-chemical properties related to hazards (e.g flammability, explosibility) and related risks (fire, explosion sensitivity, self-heating…). Dedicated experiments will focus on the wood biomass, refuse derived fuels and catalysts. The risk assessment will focus on comparison of process configurations with respect to safety (e.g. hydrogen integration and storage, reforming and CCS options), identification of accident scenarios, evaluation of ATEX zones, choice of equipment, safe handling and storage of biomass and proposition of technical and organizational safety barriers. The results will contribute to the safe development and deployment of the FLEDGED technologies.
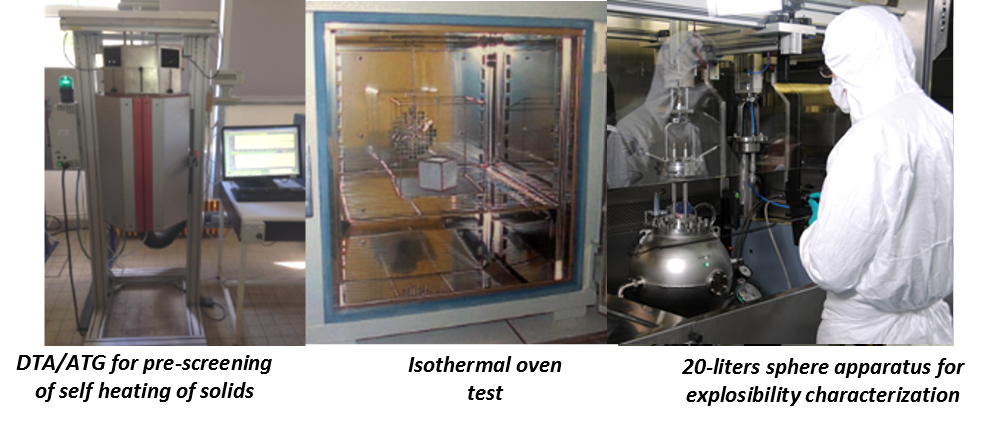
Financial analysis for commercial exploitation of FLEDGED technology (ECONWARD, FRAMES and SUMITOMO FW)
Two exploitation business models of FLEDGED technology have been conducted to produce DME from different types of biomass. The first exploitation model proposes the use of lignin and wood biomass as raw material, while the second model proposes biomass produced from Municipal Solid Waste by ECONWARD technology as feedstock. In the first exploitation model, the most important parameters affecting DME production cost are:
- Fixed Capital investment
- Biomass price
- Overall efficiency for DME production
In the first financial analysis, the obtained range of DME sales price (1100 – 1200 €/ton DME) exceeds the current commercial range of 400 – 500 €/ton DME when using lignin and wood biomass.
In the second exploitation model, the DME production cost has been analyzed using biomass from MSW as feedstock. In this model, ECONWARD technology provides a pretreatment of MSW to recover the organic fraction and produce a homogenous and sanitized biomass. The pretreatment increases CAPEX, but it allows to valorize a waste that today ends mostly in a landfill. In a waste management perspective, the process is a cost-effective solution which provides a solution aligned with the European directives in circular economy. The obtained range of DME sales price (100 – 2000 €/ton DME) is competitive under certain values of Fledged technology CAPEX and tipping fee. It is necessary to further improve the process and technology to make the exploitation models more competitive and attractive. The most realistic possibilities nowadays are:
- CAPEX reduction. Look for possible synergies (utilities) or alternative technologies that cheapen the process,
- OPEX reduction. One improvement could be a separation of ashes from sorbent in SEG process, and reuse the SEG sorbent in the process.
- Tipping fee increase. It would bring greater savings in case of using MSW as feedstock, reducing operational cost of the plant.
- DME becomes an objective fuel and Authorities decide to subsidize its production.
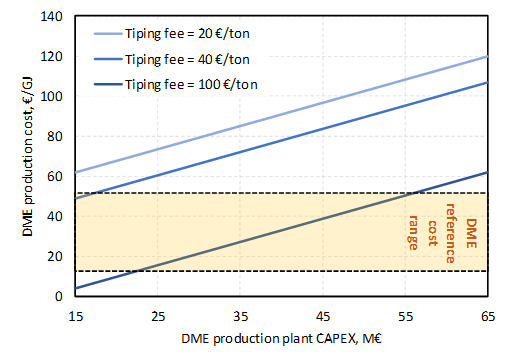
Preliminary Life Cycle Assessment of FLEDGED DME (QUANTIS)
Life cycle assessment is a technique to assess the environmental impacts associated with all the stages of a product’s life from raw material extraction to use and end-of-life. In the preliminary life cycle assessment the baseline FLEDGED scenario was assessed (sorption enhanced gasification followed by a sorption enhanced DME synthesis with wood pellets used as raw material).The net climate change impact (i.e. the sum of the greenhouse gases emitted) of FLEDGED DME is 88 g CO2-eq per 1 km driven. The surplus of electricity generated by the FLEDGED process, if sold to the market, can replace electricity production from other sources avoiding the emissions of 12 g CO2-eq/km.
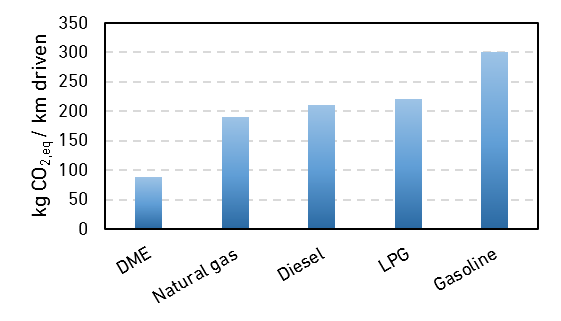
The comparison with other conventional fuels from a life cycle perspective shows that FLEDGED DME is advantageous in terms of climate change impact (54% less impact than natural gas, 58% less than diesel, 60% less than GPL and 71% less than gasoline).
Scientific publications
Reversible deactivation of γ-alumina by steam in the gas-phase dehydration of methanol to dimethyl ether (Boon J., van Kampen J., Hoogendoorn R., Tanase S., van Berkel F.P.F.,van Sint Annaland M., Catalysis Communications, Volume 119, 2019)
Abstract
Acidic γ-Al2O3 is an active catalyst for the dehydration of methanol to dimethyl ether (DME). However, the produced steam reduces the activity. In this work, the influence of the exposure of γ-Al2O3 to steam on the catalytic activity for methanol dehydration has been determined. At 250 °C and increasing stream partial pressure the conversion of γ-Al2O3 into γ-AlO(OH) is observed at a p(H2O) of 13–14 bar. As a consequence, the catalytic activity decreases, reducing the rate of methanol dehydration to around 25%. However, this conversion is reversible and under reaction conditions γ-AlO(OH) converts back to γ-Al2O3, recovering its catalytic activity.
Open access full paper on Zenodo
Steam separation enhanced reactions: Review and outlook (van Kampen J., Boon J., van Berkel F.P.F. Vente J., van Sint Annaland M., ChemicalEngineering Journal, Volume 374, 2019)
Abstract
Enhancement by steam separation is a promising process intensification for many types of reactions in which water is formed as a byproduct. For this, two main technologies are reactive vapor permeation (membrane technology) and reactive adsorption. Both can achieve significant conversion enhancement of equilibrium limited reactions by in situ removal of the by-product steam, while additionally it may help protecting catalysts from steam-induced deactivation.
In general, reactive permeation or reactive adsorption would be preferable for distinctly different process conditions and requirements. However, although some advantages of reactive steam separation are readily apparent from a theoretical, thermodynamic point of view, the developments in several research lines make clear that the feasibility of in situ steam removal should be addressed case specifically and not only from a theoretical point of view. This includes the hydrothermal stability of the membranes and their permselectivity for reactive steam permeation, whereas high-temperature working capacities and heat management are crucial aspects for reactive steam adsorption. Together, these developments can accelerate further discovery, innovation and the rollout of steam separation enhanced reaction processes.